Transition metal
ions (and metal oxide ions) have the ability to break C-C bonds in simple
hydrocarbons and to react with C-H bonds,
even in methane. We are interested in looking at the detailed mechanisms
for these reactions. We do this by using electronic and vibrational spectroscopy to characterize
the bonding, energetics and geometries of the reaction intermediates
and the charged reactants and products. The system we have studied most
extensively is the direct conversion of methane to methanol by iron
oxide: FeO++ CH4 --> Fe+ + CH3OH.
A schematic potential energy surface for this reaction is shown below.
|
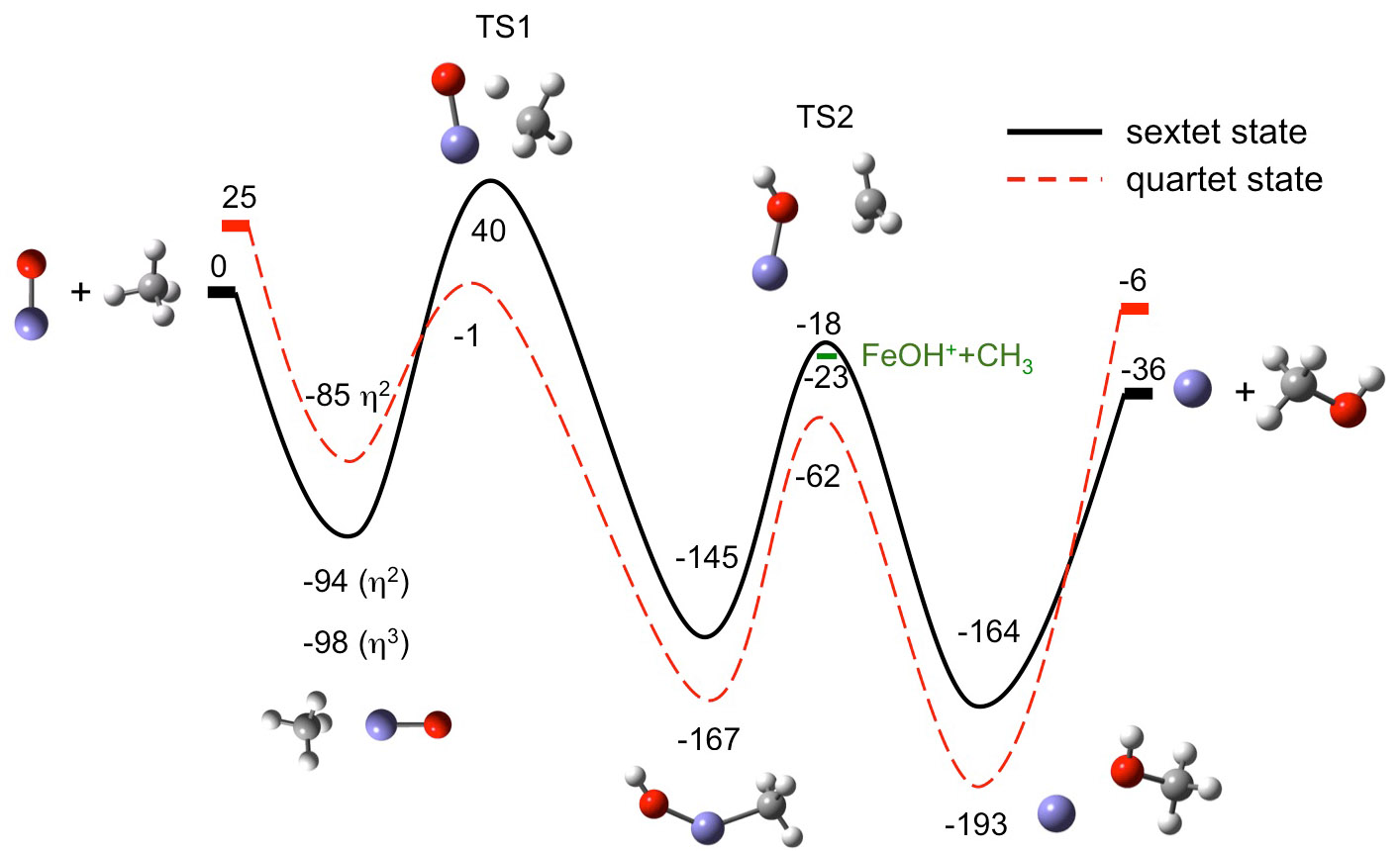 Calculated (CBS-QB3) FeO+ + CH4 Potential Energy Surface |
 Vibrational Spectra of Bare and Argon-tagged Insertion Intermediate |
|
In order to form
methanol, the reactants first form the entrance channel complex. Hydrogen abstraction leads to the critical intermediate [HO-Fe-CH3]+
. This intermediate can dissociate to form the undesirable
FeOH+ + CH3 products or it can rearrange to form
the exit channel complex, which falls apart to Fe+
+ CH3OH. We have studied the spectroscopy
of the FeO+ reactant, as well as the critical [HO-Fe-CH3]+
intermediate and the H2O...FeCH2+ intermediate,
which leads to the minor FeCH2+ + H2O
products. Vibrational spectra of the [HO-Fe-CH3]+ insertion intermediate in the C-H and O-H stretching regions are shown above.
Ions such as MCH2+
are important intermediates in reactions that couple small hydrocarbons
to make larger ones. For example, tungsten (and several other third-row
transition metals) react sequentially with methane:
W+
+ CH4 --> WCH2+ + H2
WCH2+
+ CH4 --> WC2H4+ + H2, etc.
producing ions as
large as WC8H16+. We have studied MCH2+
(M=Fe, Co, Ni, Au, Ta) and plan to extend these studies to tungsten
and platinum.